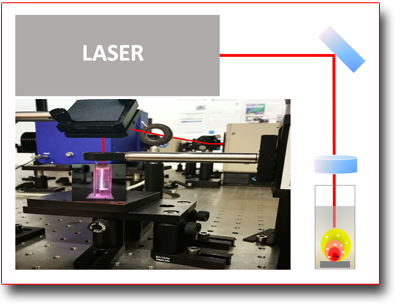
Nanoparticles synthesis by Laser Ablation in Liquid
Antonio Santagata - antonio.santagata@ism.cnr.it
LIBS-FTIR/FemtoLAB
By "ablation" one refers to the "vaporization" induced, in the form of plasma, of a solid material. The process is characterized by the complex interaction mechanism that is generated by focusing a high power laser beam onto the surface of a target material. The generation and expansion of the plasma in liquid involves firstly the formation of a shock wave and then of a cavitation bubble. This bubble, whose expansion is limited by the surrounding liquid, is confined and compressed until collapsing and oscillating with the formation of new damped cavitation bubbles. During the cavitation bubbles collapse repeated coalescence phenomena of the "vaporized" species is involved which give rise to colloidal solutions of nanoparticles (NPs) of the ablated material. The NPs, which are negatively charged, have high stability even in the absence of surfactants and can often have surface layers (e.g. core shell) of oxides or other components coming from the solvent or other components on purpose added to the solution. The parameters that control the process are connected both to the pulsed laser used (e.g. pulse duration) and to the type of liquid, or type of solution, which, the species produced after the collapse process of the cavitation bubble, can continue to react.
The LAL activities are also carried out in collaboration with the Physical-Chemistry Laser Laboratory of the University of Basilicata which expands the offer by the availability of other experimental equipments and techniques for characterizing the obtained NPs (e.g. HR-TEM).
TECHNICAL SPECIFICATIONS
- Spectra Physics Ti:Sa “fs” Laser
Spitfire Pro - Regenerative Amplifier (120 fs; 1kHz; 4 mJ @ 800 nm; SH: 1.5 mJ @ 400 nm) - Quanta System Nd:YAG “ns” Laser
Prototype (7 ns; 10 Hz; 100 mJ @ 532 nm) - Time resolved shadowgraphy
- Andor iStar “Inductively Charge Couple Device – ICCD” camera (t ≥ 2 ns; Spectral range = 250-900 nm, Pixeldim = 13 μm x 13 μm)
AVAILABLE TECHNIQUES
-
Production of colloidal solutions of nanoparticles
-
Expansion dynamics of the cavitation bubbles and shock waves by shadowgraphy
SAMPLES
- Flat samples with lateral dimension 10 mm x 10 mm (minimum) and 15 mm x 25 mm (maximun); thickness 15 mm (maximun).
USED FOR
-
Catalysts
-
Biosensors
-
Colorimetric sensors
-
Biomedical applications
-
Optoelectronics
-
Nanocomposites
CASE STUDIES
Generation of Nanodiamonds
During the collapse of the cavitation bubbles of the LAL process both high temperatures (thousands of K) and high pressures (GPa) can be reached. These parameters play a relevant role within the condensation process of the ablated species which can lead to the formation of metastable phases. For instance, nanodiamonds can be formed through simple ablation of graphite submerged in water by employing fs laser pulses. However, in order to obtain nanodiamonds, a proper laser repetition rate should be employed. As a matter of fact, the nanoparticles produced by LAL can change their properties as a function of the fs pulsed laser repetition rate used, which, as shown with Raman spectroscopy, switch, from diamond-like carbon (DLC), nanoparticles (10-100 Hz) to nanodiamonds (1 kHz)
See: Antonio, Santagata et al. J. Phys. Chem. C 115, 5160 (2011)
DOI: 10:1021/jp1094239
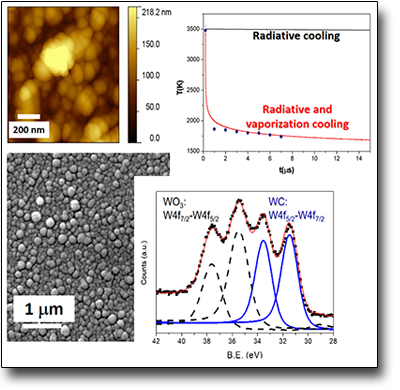
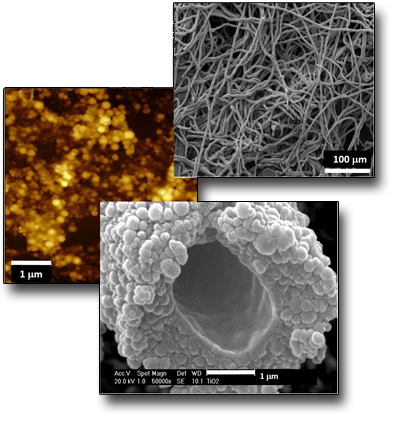
Microtubes formation by selfassembling of NPs
The ablation by fs pulses of metallic Ti submerged in water, leads to the formation of Ti species having different oxidation states, that means the co-presence of Ti2O3, TiO2 and TiOH NPs in which rutile (TiO2)represents the crystalline phase. Although the NPs dimensions are in the range of 5-100 nm, the most probable ones have diameters between 5-10 nm. The main feature of the ablation process induced by fs laser pulses performed in these conditions is, however, characterized by the presence of nanoscopic lamellae which, together with the NPs, give rise, after a few days of permanence in solution, to the formation of nanofilaments of length and thickness of tens and a few nanometers, respectively. The permanence of these nanostructures in solution (e.g. > 7 days) induces the spontaneous formation of microtubes having length and diameter of the internal wall of some mm and 2 µm, respectively. The mechanism of microtube formation, onto which the NP aggregate by coalescence, it is related the presence of TiOH species and the whole chemistry induced in solution by the LAL process itself.μm.
See: Angela, De Bonis et al. Surf. Coat. Tech. 207, 279 (2012)
DOI: 10.1016/j.surfcoat.2012.06.084
Silver-silica core-shells nanocomposites prepared from nanoporous silica and silver target by ultra-short pulsed laser ablation in liquid
Hexagonally ordered mesoporous silica materials, MCM-41 and SBA-15, have been synthesized and loaded with Ag nanoparticles (Ag/SBA-15 and Ag/MCM-41), utilizing both chemical synthesis and ultra-short pulsed laser ablation in liquid. All preparations have significant amount of 5–50 nm size silver agglomerates on the outer surface of the silica particles. The laser ablation process did not cause significant destruction of the SBA-15 structure and metallic silver (Ag0) nanoparticles were mainly generated. Moreover, it has been also evidenced by us also the formation of stable silver-silica core-shell (Ag@SBA-15 and Ag@MCM-41) nanoparticles by direct laser ablation of a Ag target submerged in a static colloidal solution of MCM-41 or SBA-15 silica nanoporous materials. The outcomes show that the choice of the mesoporous silica material can affect the silica shell thickness in addition to the Ag NPs size distribution.
See:
- Ágnes, Szegedi, et al., Appl. Phys. A 117, 55 (2014) DOI: 10.1007/s00339-014-8499-8
- Antonio, Santagata, et al., J. Phys. D: Appl. Phys. 48, 205304 (2015)
- DOI: 10.1088/0022-3727/48/20/205304
Contact: Ambra Guarnaccio - ambra.guarnaccio@ism.cnr.it
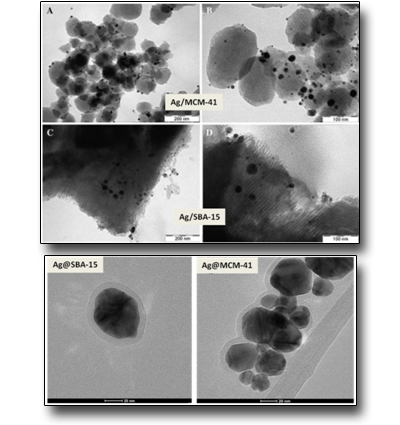