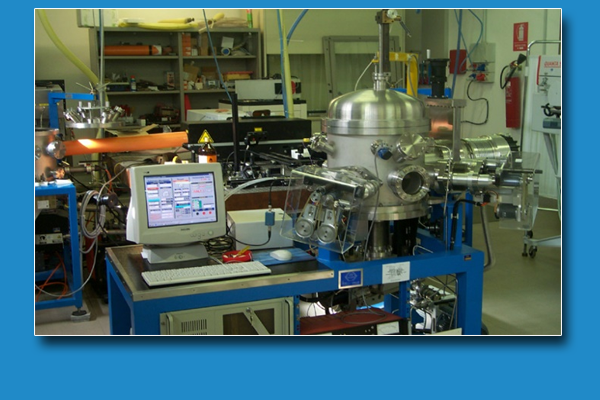
TECHNICAL SPECIFICATIONS
PLD
- Nd-Yag laser (Time= 10 nanosec, Frequency= 10 Hertz)
- X-Ray diffraction (Siemens D5000)
- Total X Ray Fluorescence (Oxford-Si-Pin)
MOKE
- longitudinal geometry
- He-Ne laser (632 nm)
- magnetic field 800 G
AVAILABLE TECHNIQUES
- PLD
- XRD
- TXRF
- Longitudinal MOKE
SAMPLE
MOKE
-
thin films
-
nanoparticles deposited and/or grown on substrates that do not have magnetic properties
USE FOR
-
Semiconduttori Organici/Inorganici;
-
Thin films/coatings;
-
Nanoparticelle.